For 70 years after the publication of the Origin of Species, it seemed as if Lamarck‘s shadow would loom forever over Darwin. On the one hand, most biologists came to the reality of evolution — that living species shared a common ancestry and had been transformed over time. But natural selection — the engine of evolution, according to Darwin — remained controversial. Many biologists argued that there must be some built-in “direction” to the variation that arose in each generation, helping to push each lineage towards its current state.
Many of these first geneticists who rediscovered Mendel‘s insights around 1900 also opposed natural selection. After all, Darwin had talked of natural selection gradually altering a species by working on tiny variations. But the Mendelists found major differences between traits encoded by alleles. A pea was smooth or wrinkled, and nothing in between. In order to jump from one allele to another, evolution must make giant jumps—an idea that seemed to clash with Darwin.
Natural selection in a Mendelian world
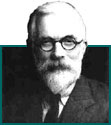
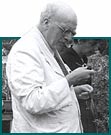
But in the 1920s geneticists began to recognize that natural selection could indeed act on genes. For one thing, it became clear that any given trait was usually the product of many genes rather than a single one. A mutation to any one of the genes involved could create small changes to the trait rather than some drastic transformation. Just as importantly, several scientists — foremost among them Ronald Fisher (above left), JBS Haldane (above right), and Sewall Wright (below left) — showed how natural selection could operate in a Mendelian world. They carried out breeding experiments like previous geneticists, but they also did something new: they built sophisticated mathematical models of evolution.
Small, not drastic, changes
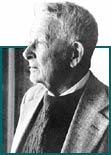
Known as “population genetics,” their approach revealed how mutations arise and, if they are favored by natural selection, can spread through a population. Even a slight advantage can let an allele spread rapidly through a group of animals or plants and drive other forms extinct. Evolution, these population geneticists argued, is carried out mainly by small mutations, since drastic mutations would almost always be harmful rather than helpful.
Wright introduced the most compelling metaphor in population genetics, known as the “adaptive landscape” (see figure, below). You can imagine the varying fitness of different combinations of genes as a hilly landscape, in which the valleys represent less-fit combinations of genes and the peaks represent the fitter ones. Natural selection tends to move the populations towards the peaks of the hills. But since the environment is always changing, the peaks shift, and the populations follow after them in a never-ending evolutionary journey.
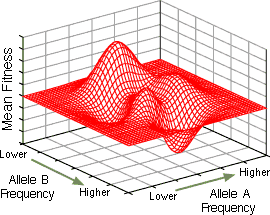
Natural selection in the wild
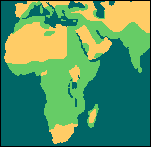
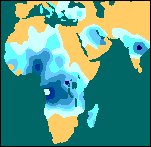
Population genetics became one of the key elements of what would be called the Modern Synthesis. It showed that natural selection could produce evolutionary change without the help of imaginary Lamarckian forces. Scientists have used the mathematical tools developed by Fisher, Wright, and Haldane to measure evolutionary change in the wild with exquisite precision. Their insights have even allowed medical researchers to decipher the puzzle of some hereditary diseases. Sickle-cell anemia, for example, is caused when children inherit two defective copies of a gene involved in making hemoglobin. But a single copy of this allele can give some protection against malaria (see figures, right). Natural selection finds a balance between the reproductive disadvantage of being born with two copies of the allele and the advantage of having one. Genetic disorders such as sickle-cell anemia are actually the agonizing byproduct of natural selection acting on our ancestors.