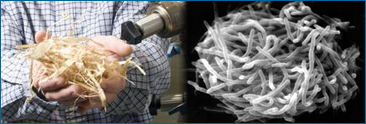
In celebration of the Year of Science’s April theme, energy resources, we bring you a story from the frontiers of energy research that depends on evolution. Right now, most of us fill up our gas tanks with fossil fuels, the remains of plants and animals that died many millions of years ago and eventually became petroleum — but, of course, this can’t last forever. Petroleum is a limited resource and will eventually run out. To help solve this problem, many scientists, policymakers, business people, and concerned citizens have placed their hopes in biofuels — fuel derived from plant matter that we can grow today. Biofuels might sound like an ideal solution to our energy problems, but there are still major kinks to work out — kinks that evolution can help us overcome.
Where's the evolution?
Part of the problem with the biofuels currently produced in the US is that they are mainly made from corn — a greedy crop that sucks nutrients from the soil and needs lots of fertilizers (which are often themselves derived from fossil fuels!). Even worse, since corn is also a food crop, using it to produce biofuel drives up the cost of this dietary staple. Furthermore, a tiny fraction of the plant, the kernels, can be used to make fuel. Biofuel production would be much more efficient if it used whole plants and if it could rely on less greedy plants that are not also used as food. Such a fuel might be a viable alternative to gasoline. Unfortunately, getting biofuel out of plant matter is no easy task; it requires a series of complex chemical reactions. Figuring out how to make those reactions happen without using up tons of energy in the process is the job of synthetic biologists — and they have turned to evolution to help crack this nut.
Evolution in the wild involves the survival and reproduction of organisms with traits particularly well-suited to their environments. Synthetic biologists, like Jay Keasling of UC Berkeley, Frances Arnold of Caltech, and many others, are trying to replicate this process in the lab — only instead of evolving organisms with particular traits, they aim to evolve molecules that are particularly good at transforming plant matter into fuel. The process is called directed evolution, and it works like this:
- Begin with a gene that does something close to the job you have in mind. Perhaps it produces a molecule that can chop up cellulose — the substance that makes up most plant matter and the most abundant organic molecule on Earth.
- Produce many copies of the gene with slight variations. Often, this is done by intentionally using a “bad” copying system — a process for copying the DNA that introduces lots of random mutations. You’ll end up with a pool of similar genes with slight genetic differences from one another.
- Insert the different gene copies into organisms — usually bacteria or yeast. This is done to find out what all the different gene versions do. Bacteria and yeast have all the cellular machinery needed to turn on the gene and let us see how it functions.
- Screen the organisms to see which are best at performing the job you have in mind. In this case, you might be looking for the ones that are speediest at breaking down cellulose. As in evolution in the wild, most of the new mutations will be detrimental or neutral — that is, they will make the molecule worse at its job or will have no effect on it at all. However, if you look at enough mutants, you are likely to find a few that improve the molecule’s functionality.
- Select the best gene version and use it in Step 1. Repeat this cycle many times. It’s unlikely that any one mutation would make a huge difference in the functioning of the molecule — but by selecting the best gene from each generation and using it as the basis for the variants produced in the next generation, you can identify a series of changes that, together, make a big improvement.
This process mimics evolution by natural selection. In natural selection, the organisms with the genes that perform best in a particular environment survive to pass those genes (possibly with new mutations) on to the next generation. As this cycle is repeated over many generations, organisms with genes for traits well suited to the environment evolve. Directed evolution works the same way, but the selection is focused on gene versions that are good at doing a certain job. The key difference between the two processes is that, in the wild, the environment determines which gene versions are most successful, and in the lab, the scientist does. While natural evolution has no goal, directed evolution can be channeled toward the ends we have in mind.
Of course, the end that we have in mind — transforming plant material into fuel — will be easier to achieve if we start with genes that already have some of the functions we want. Genes like this do exist. After all, plant matter doesn’t accumulate indefinitely in our gardens or on the forest floor. It is eventually broken down, mainly by organisms like microbes and fungi. While such life forms might not seem very exciting — just compare the number of nature documentaries on mushrooms to the number on meerkats — for synthetic biologists, these organisms are treasure troves of thrilling new genes that could spark a biofuels revolution.
Directed evolution is a key tool for synthetic biologists because those new genes (currently hidden away in bacteria on the rainforest floor or in the gut of an exotic termite) won’t be perfectly matched to our biofuels needs. That mismatch can be chalked up to the genes’ own evolution. Evolution shaped these genes in their complex natural environments — environments in which optimizing one trait often meant downgrading another. For example, improving a molecule’s efficiency at chopping up cellulose might also mean putting more energy into producing the molecule or making the molecule less stable at different temperatures. Nature selection balances such trade-offs and tends to maximize the overall survival and reproduction of organisms — not the efficiency of particular molecules. The genes we find in nature are the product of a balancing act among evolutionary trade-offs and constraints — which means that they work well enough in the real world but probably aren’t ideal for the mass production of biofuel. One of the advantages of directed evolution is that, in the lab, scientists can remove these trade-offs and constraints, optimizing the genes for performance in a particular environment.
The alternative to optimizing genes through evolution is improving them through engineering — intentionally making particular changes in a gene for certain effects. We definitely have the technology to alter genes — in fact, these days, you can order a particular genetic sequence online and have the DNA shipped directly to you! The problem is that in few cases do we have enough knowledge to figure out exactly which changes will produce the desired results. That’s why directed evolution is such an appealing way to attack the problem of building better biofuels. Evolution, after all, has no foresight — so when scientists use directed evolution, they don’t need detailed knowledge of which genetic changes will help solve their problem. By screening millions of variants and building on the best of those, directed evolution lets science stumble onto engineering solutions that we might never have thought of — solutions that just might allow us to unlock the tantalizing, but still unrealized, promise of biofuels.
Primary literature:
- Arnold, F. H. (2008). The race for new biofuels. Engineering & Science 71(2):12-19.
- Tollefson, J. (2008). Energy: Not your father's biofuels. Nature 451:880-883.
- Lee, S.K., Chou, H., Ham, T.S., Lee, T.S., and Keasling, J.D. (2008). Metabolic engineering of microorganisms for biofuels production: From bugs to synthetic biology to fuels. Current Opinion in Biotechnology 19(6):556-563. Read it »
News articles:
- A description of the role of directed evolution in biofuel development from Lawrence Berkeley National Laboratory
- A friendly explanation of why it's so hard to get fuel out of plant matter from the Great Lakes Bioenergy Resource Center
- A more technical article on the challenges and approaches to biofuel production from Engineering and Science
Understanding Evolution resources:
- A tutorial on natural selection
- A research profile on a scientist who uses directed evolution to reshape molecules
- A case study on evolutionary constraints
Background information from Understanding Global Change:
- Review the concept of natural selection. Explain how each of the four steps described on that page relate to the process of directed evolution described above.
- In the process of directed evolution, the gene of interest is mutated randomly. Despite this randomness, the process ends up producing a gene with a much improved function. Explain how random mutations can end up producing non-random change.
- The article above explains why the ideal genes for biofuel production are not found in wild organisms. In your own words, explain why this is the case.
- The article above describes how directed evolution is being used to improve the process of biofuel production. Research and describe another application of directed evolution. Be sure to explain what the target of selection is and what trait is being focused on.
- Teach about how natural selection acts on mutations: In this classroom activity for grades 9-12, students build, evolve, and modify paper-and-straw "birds" to simulate natural selection acting on random mutations.
- Teach about applications of evolutionary theory: In this article for grades 9-12, evolutionary biologist Jim Bull gives his perspective on how evolution matters to society today, including a section on directed evolution.
- Teach about evolutionary constraints: In this interactive investigation for grades 6-12, students delve into the amazing world of the arthropods to examine their success and their evolutionary constraints.
- Arnold, F. H. (2008). The race for new biofuels. Engineering & Science 71(2):12-19.
- Lawrence Berkeley National Laboratory. (2009). The evolutionary road to biofuels. Year of Science series. Retrieved March 23, 2009 from the Lawrence Berkeley National Laboratory.
- Tollefson, J. (2008). Energy: Not your father's biofuels. Nature 451:880-883.