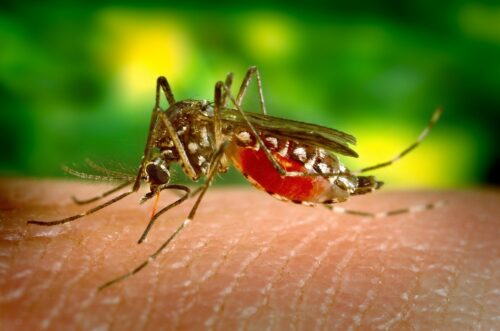
A recent outbreak of the Zika virus in the Americas has brought mosquitoes buzzing onto the front pages of newspapers. With a bite, infected mosquitoes can transmit Zika to humans. While most adults will experience only mild symptoms from the virus, the results can be devastating for a fetus carried by an infected mother. Evidence suggests that the Zika virus is likely responsible for a surge of birth defects in Brazil, and nearby countries are on high alert as the virus spreads. Of course, Zika is in the news now, but mosquito-borne diseases are nothing new. Malaria, in particular, threatens human health on an even wider scale than Zika. The malaria protozoan kills hundreds of thousands each year despite our efforts to snuff it out. Campaigns to fight malaria have tackled the problem on many fronts — treating the disease with new drugs, helping people protect themselves from insect bites, and reducing mosquito populations. With advances in genetic technology, scientists are investigating a new approach: genetically engineering mosquitoes to resist the malaria parasite. In fact, in 2012, researchers showed that mosquitoes engineered to carry two different malaria resistance genes, could not transmit the disease at all! But in order to actually combat malaria with this exciting discovery, we’d need to figure out how to get the resistance genes to spread through mosquito populations…
Where's the evolution?
The spread of malaria resistance genes through a mosquito population is fundamentally a question of microevolution — a change in gene frequency from one generation to the next. Scientists have been trying to figure out how to cause mosquito populations to evolve over the course of generations such that mosquitoes carrying the engineered resistance genes would become more and more frequent, and normal, malaria-carrying mosquitoes would become less frequent. When populations evolve via natural selection, a gene spreads because it has a selective advantage, that is, because it gives a boost to the survival or reproduction of individuals carrying that gene. But because malaria doesn’t seem to harm mosquitoes infected with it, a gene to resist malaria (while a boon for human survival), has no benefit at all for mosquitoes. So natural selection would not, by itself cause the resistance genes to spread.
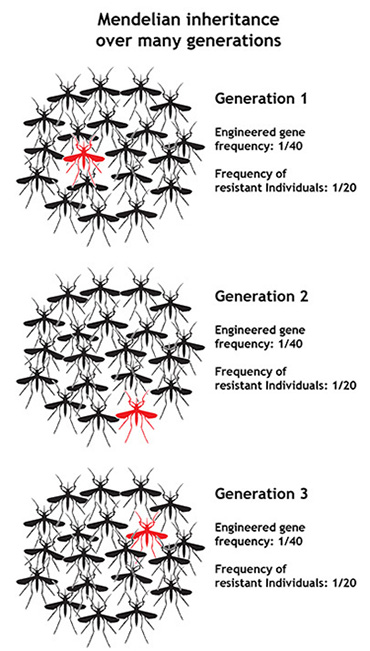
When a gene does no particular harm or particular good, as seems to be the case for malaria resistance genes in relation to mosquitoes, evolutionary theory tells us what is likely to happen to the frequency of that gene in the short term: it will stay about the same. This happens because of basic Mendelian inheritance — the idea that genes are inherited as discrete units and that we pass the genes that we inherit on to our offspring in an unbiased way. It works like this: Humans, mosquitoes, and many other organisms carry two copies of each gene. If an individual carrying one mutant version of a gene and one normal version breeds with an individual that carries two normal versions, on average only half of their offspring will carry the mutant gene version. In this way, over time, the frequency of the mutant gene stays about the same — and if it starts off as a rare gene, it remains a rare gene. This is a big hurdle for researchers trying to shape evolution through genetic engineering. In order to have an impact on a population, scientists would have to produce a large number of engineered individuals.
Now, new research suggests a way to get over that hurdle: a process called gene drive, which biases inheritance so that certain genes are passed along more frequently than others. A few months ago, biologists announced that they’d successfully applied this idea to malaria resistance in mosquitoes. The process relies on a mechanism that converts a heterozygote (an individual carrying one copy of a particular gene version) into a homozygote (an individual carrying two copies of that gene version). The scientists injected a specially engineered piece of DNA made up of a suite of genes into mosquito embryos. This DNA contained the two genes for malaria resistance, as well as a gene that acts as molecular scissors by producing a DNA-cutting protein. Inside the mosquito cell, this new stretch of DNA inserts itself into one mosquito chromosome at a particular location. When that section of the chromosome is expressed or activated, it produces both the proteins that help the mosquito resist malaria as well as a protein that cuts the corresponding (i.e., homologous) chromosome at the exact same location that the new DNA was inserted. When the cell repairs break, the cellular machinery automatically uses the corresponding chromosome (the one carrying the engineered DNA) as a template, so the resistance genes, as well as the DNA-cutting mechanism, are copied to the second chromosome. And when that cell divides as the embryo grows into an adult mosquito, the chromosomes carrying the engineered DNA are copied along with the rest of the genome.
With this so-called “super-Mendelian” inheritance, genes that do no particular harm or good can easily spread through populations. This is because when one of these genetically engineered mosquitoes mates with a wild mosquito (which carries no malaria resistance genes), all of the offspring (not just half) will carry the resistance genes. And theoretically, we’d expect those offspring to go off, mate with other wild, susceptible mosquitoes, and produce all resistant offspring as well. When researchers tested this idea in the lab, they found that the biased spread of the resistance genes occurred quite efficiently for several generations. If such a system could be successfully deployed in areas where malaria transmission is common, it could cause infection rates to drop precipitously over time — mosquito generation by mosquito generation.
Of course, we are not yet ready to attack malaria with super-Mendelian mozzies. The recently developed technique seems to eventually break down in lineages descended from genetically modified females — though scientists are working to fix this problem. Further research will also be needed to make sure that the system is effective where it matters: in the field. And importantly, scientists must investigate the potential risks of attempting this sort of intervention on a large scale. After all, at its heart, a gene drive system is designed to change the course of a species’ evolution forever. Unleashing these new genes is a bit like releasing a new species into the wild. In the past, invasive species and biological control agents have sometimes had disastrous consequences for local ecosystems, so scientists want to be confident that releasing genetically engineered mosquitoes will not cause a ripple of negative effects. Genetically driving evolution to our desired end represents a tantalizing strategy for combating insect-borne diseases like malaria and Zika virus without use of drugs or pesticides — but since we can’t control the genetic genie in this bottle once she is out, scientists are erring on the side of caution and keeping the modified mosquitoes in the lab until we have more information about the long term consequences of this strategy.
News update, August 2016
With the spread of the Zika virus to the United States a few weeks ago, mosquito-borne illnesses continue to appear in headlines…just as scientists attempt to expand research efforts that might stop some of them. In June, the National Academies of Sciences, Engineering, and Medicine announced that it was endorsing additional research into gene drive technology despite the significant risks it poses. The advisory group concluded that the potentially major benefits of the research outweigh the possible harm it might cause and even outlined an approach to running field tests. Since the support of the National Academies could spur additional funding for research in this area, we’ll be on the lookout for breaking news and pass it on to you right here!
News update, August 2018
In the two years since we first reported on gene drives here, scientists have continued to research how this genetic tool might be deployed to address public health crises — as well as how it might fail. In fact, one of the biggest roadblocks to successfully using gene drives to push the evolution of disease vectors in directions that benefit human health seems to be evolution itself, in particular the evolution of resistance to gene drives. Researchers recently showed how the basic mechanism of a gene drive can cause mutations for resistance within a single generation. Gene drives work because of molecular “scissors” that recognize a particular location on the genome that is then repaired. However, mistakes can easily occur in that repair process that make that site (and the corresponding site on all descendent chromosomes) unrecognized by the molecular “scissors” of the drive — and without that initial cut, the new gene cannot spread. Other experiments showed that such mutations (ones that mask the intended location of the cut) spread quickly when favored by natural selection (as occurs when the gene drive is designed to reduce the fertility of the carrier) and can overwhelm the power of a gene drive, ultimately reducing its frequency in the population. Still other work has shown that such masking mutations are likely already present in the pest populations we might target with gene drives. This new research highlights how many challenges we still have to overcome before it makes sense to release a gene drive into the wild, as well as what sorts of drives are least susceptible to the evolution of resistance. All of the mechanisms described above act most strongly when the gene delivered by a drive decreases the fitness of the carrier. Hence, the drives most likely to succeed are ones that cause the spread of a mutation that has little impact on the carrier (i.e., a gene that causes a mosquito to be resistant to the malaria parasite), but a large impact on human health.
Primary literature:
- Gantz, V.M., Jasinskiene, N., Tatarenkova, O., Fazekas, A., Macias, V.M., Bier, E., and James, A.A. (2015). Highly efficient Cas9-mediated gene drive for population modification of the malaria vector mosquito Anopheles stephensi. Proceedings of the National Academy of Sciences. 112: E6736-E6743 Read it »
- National Academies of Sciences, Engineering, and Medicine. (2016). Gene Drives on the Horizon: Advancing Science, Navigating Uncertainty, and Aligning Research with Public Values. Washington, DC: The National Academies Press. DOI: 10.17226/23405. Read it »
News articles:
- A blog post about how gene drives work from Scientific American
- An article on gene drive in mosquitoes from the New York Times
- More on gene drives from ScienceNews
Understanding Evolution resources:
- When a new gene version arises in a population (through either mutation or genetic engineering), when would we expect its frequency to increase via natural selection? When would we expect it to stay about the same? When would we expect it to decrease via natural selection?
- The spread of malaria resistance genes would be highly advantageous for human populations. Would we expect these genes to spread through mosquito populations on their own? Explain why or why not.
- Is gene drive a way of changing the action of natural selection? Why or why not?
- Would you have concerns about releasing genetically engineered mosquitoes into the wild, or would you expect the benefits to outweigh the risks? Explain your answer.
- Advanced: If the gene drive system described here worked perfectly in the wild, at what rate would you expect the resistance genes to spread through a mosquito population? If the resistance genes have a frequency of 1 in 40 in Generation 1, in what generation number would you expect the genes to reach a frequency greater than 90%?
- Advanced: As described above, the malaria parasite has a nontrivial impact on human health. Do some research online to find out if this impact is strong enough to cause natural selection in human populations. Describe your findings and the evidence that supports your conclusion.
- Teach about Mendelian and population genetics: This set of PowerPoint slides featuring questions for problem-based discussion (i.e., open-ended questions that engage students with each other and with course material) can be easily incorporated into lectures on populations genetics. They are appropriate for college-level courses.
- Teach about evolution by natural selection: This classroom exercise from the journal Evolution: Education and Outreach is for grades 9-16 and introduces the concept of evolution by natural selection in a hypothesis-driven, experimental fashion, using a deck of cards.
- Burt, A. (2003). Site-specific selfish genes as tools for the control and genetic engineering of natural populations. Proceedings of the Royal Society of London, B. 270: 921-928.
- Champer, J., Reeves, R., Oh S. Y., Liu, C., Liu, J., Clark, A. G., and Messer P. W. (2017). Novel CRISPR/Cas9 gene drive constructs reveal insights into mechanisms of resistance allele formation and drive efficiency in genetically diverse populations. PLOS Genetics. 13: e1006796.
- Drury, D. W., Dapper, A. L., Siniard, D. J., Zentner, G. E., and Wade, M. J. (2017). CRISPR/Cas9 gene drives in genetically variable and nonrandomly mating wild populations. Science Advances. 3: e1601910.
- Gantz, V.M., Jasinskiene, N., Tatarenkova, O., Fazekas, A., Macias, V.M., Bier, E., and James, A.A. (2015). Highly efficient Cas9-mediated gene drive for population modification of the malaria vector mosquito Anopheles stephensi. Proceedings of the National Academy of Sciences. 112: E6736-E6743.
- Hammond, A. M., Kyrou, K., Bruttini, M., North, A., Galizi, R., Karlsson, X., … Nolan, T. (2017). The creation and selection of mutations resistant to a gene drive over multiple generations in the malaria mosquito. PLOS Genetics. 13: e1007039.
- Harmon, A. (June 8, 2016). Panel endorses 'gene drive' technology that can alter entire species. The New York Times. Retrieved August 4, 2016 from the New York Times (article)
- Isaacs, A.T., Jasinskiene, N., Tretiakov, M., Thiery, I., Zettor, A., Bourgouin, C., and James, A.A. (2012). Transgenic Anopheles stephensi coexpressing single-chain antibodies resist Plasmodium falciparum development. Proceedings of the National Academy of Sciences. 109: E1922-E1930.
- Isaacs, A.T., Li, F., Jasinskiene, N., Nirmala, X., Marinotti, O., Vinetz, J.M., and James, A.A. (2011). Engineered resistance to Plasmodium falciparum development in transgenic Anopheles stephensi. PLoS Pathogens. 7: e1002017.
- Ledford, H. (2015). Caution urged over editing DNA in wildlife (intentionally or not). Nature. 524: 16.
- Ledford, H., and Callaway, E. (November 23, 2015). 'Gene drive' mosquitoes engineered to fight malaria. Nature. Retrieved March 28, 2016 from Nature (http://www.nature.com/news/gene-drive-mosquitoes-engineered-to-fight-malaria-1.18858).
- Sarkar, S. (2018). Researchers hit roadblocks with gene drives. BioScience. 68: 474-480.