Radioisotopic dating is a key tool for studying the timing of both Earth’s and life’s history. This suite of techniques allows scientists to figure out the dates that ancient rock strata were laid down — and hence, provides information about geologic processes, as well as evolutionary processes that acted upon the organisms preserved as fossils in interleaved strata.
Radioactive decay
Radioisotopic dating relies on the process of radioactive decay, in which the nuclei of radioactive atoms emit particles. This releases energy (in the form of radiation) and often transforms one element into another. For example, over time, uranium atoms lose alpha particles (each made up of two protons and two neutrons) and decay, via a chain of unstable daughters, into stable lead.
Although it is impossible to predict when a particular unstable atom will decay, the decay rate is predictable for a very large number of atoms. In other words, the chance that a given atom will decay is constant over time. Decay rates are measured in half-lives — the amount of time in which half of a radioactive element will decay. For example, as shown at left below, uranium-235 has a half-life of 704 million years. That means that in 704 million years, one gram of uranium will be reduced to ½ gram of uranium. And in the next 704 million years, it will decay leaving behind ¼ gram, and in the next 704 million years, it will decay leaving behind ⅛ gram and so on. At the same time, the amount of the element that it decays into (in this case lead-207), will increase accordingly, as shown below.
Now imagine that you have a rock sample that contains 39% uranium-235 and 61% lead-207. How old would you hypothesize the rock is? Study the graph at left above. At what point on the graph would you expect the ratio of uranium to lead to be about 39 to 61? At around 1000 million years (i.e., one billion years), as shown on the graph at right above. Thus, you would calculate that your rock is about a billion years old. Scientists usually express this as an age range (e.g., one billion years plus or minus half a million years), meaning that they are very confident that the true date falls somewhere within that range. With modern techniques, these ranges have gotten narrower and narrower, and consequently, even very ancient rocks can be dated quite precisely. For practice, use the graph above to estimate the age of a rock sample that contains 10% uranium and 90% lead.
The example above describes uranium/lead decay, which happens very slowly; however, different radioactive elements have different half-lives. Some are much shorter. This allows scientists to date events that are more or less ancient. For example, carbon-14 decays into nitrogen-14 and has a half-life of just 5,730 years. Hence, carbon-14 dating can only be used to estimate much younger ages, up to around 60,000 years. Slightly different dating techniques are used with different radioactive elements, but the same basic logic of estimating backwards based on radioactive decay remains the same.
The geology behind radioisotopic dating
Though the basic logic behind radioisotopic dating relies on nuclear physics and quantum theory, many geologic processes also factor into our ability to date a particular rock. How do scientists find the right rocks for dating? How do they know that the rock isn’t contaminated with elements that would throw off the dating? How can the formation of a rock be correlated with a particular ancient event? The answers to all of these questions lie in our understanding of the geologic processes that affect the deposition of radioactive elements. To see how it works, we’ll start at the beginning, using uranium as an example:
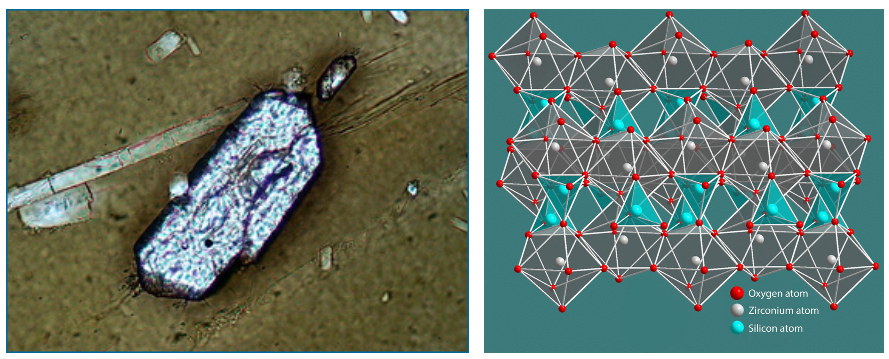
Tens to hundreds of thousands of years before a major volcanic eruption, magma builds up beneath the surface of the Earth. In the magma, crystals of zirconium silicate (called zircons), as well as other crystals, form. These zircon crystals are tiny — just a tenth of a millimeter long — but they are the key to uranium-lead dating. If these crystals were pure, they would contain just zirconium, silica, and oxygen; however, uranium happens to have a similar arrangement of outer electrons to zirconium, and so as zircons form, “mistakes” are sometimes made, and uranium is substituted for zirconium. Because lead (the stable daughter of uranium) has a very different arrangement of electrons, it does not make its way into the crystal as it is forming. The formation of crystals in the magma marks the moment that the radio-isotopic clock starts ticking. When the eruption occurs, zircons are released in the ash and lava, which then become rocks like rhyolite. Geologists hunt for these particular sorts of rock to date the volcanic eruption in which the rock formed. Zircons are nearly perfect clocks because we can be relatively certain that when the crystal formed, no lead was present — and that means that when we discover ancient zircons in rocks today, we can be relatively confident that any lead present is the result of radioactive decay.
Geologists extract the appropriate minerals from the rock (in this case, zircon crystals) and use a technique called mass spectrometry to figure out the relative amounts of uranium and lead in the zircon. Thus, when a geologist dates a rock using uranium-lead dating, he or she is actually getting an estimate on the age of its zircon crystals, which formed “shortly” before the volcanic eruption. Of course, in this case “shortly” is meant in terms of geologic timescales. The zircon formation may have occurred tens to hundreds of thousands of years before the eruption and deposition. However, when dealing with rocks that are hundreds of millions of year old, the time between zircon formation and eruption really is short in comparison.
The amount of material involved in these estimates is small, but can be used to generate powerful results. A grapefruit sized piece of granite may contain a thousand zircons — just a few milligrams of material — and only a tiny amount of each crystal (a few hundred parts per million) is actually uranium or lead and useful in dating the rock. Nevertheless, with modern techniques, scientists can measure these amounts very precisely. Furthermore, to gain confidence in their estimates, geologists date five to ten zircon crystals from the same rock. Normally, these crystals will all point to the same 200,000 year window. Occasionally, an outlying crystal will date to a much earlier time period than the others from the sample, and in these cases, geologists know that the rock sample has been contaminated by zircons from a different eruption. Furthermore, other radio-isotopic systems can be used as independent lines of evidence to validate the results from the uranium-lead method. Using such techniques, scientists can very accurately date ancient volcanic events and can extrapolate from these dates to learn about the ages of other rock strata.